德國picotweezers品牌,高分辨率三維單細(xì)胞單分子力學(xué)捕獲分析光鑷系統(tǒng)(3D Force Sensitive Optical Tweezers)
PicoTweezers是一種結(jié)合了光鑷技術(shù)及微視圖像計(jì)算集合的分子生物力學(xué)分析系統(tǒng)。PicoTweezers作為一個(gè)獨(dú)立的系統(tǒng),可以與蔡司Axiovert、AxioA1或D1顯微鏡聯(lián)合使用。PicoTweezers配備了功率為1W或5W的紅外光纖激光器,可達(dá)激光陷阱捕獲力范圍是400pN——2nN。PicoTweezers的3D-壓電平臺可以實(shí)現(xiàn)x軸和y軸為200μn;m的分辨率,在z軸方向可以實(shí)現(xiàn)20μn;m的分辨率。獨(dú)特的視頻分析系統(tǒng)(Video-analysis)可以達(dá)到至少2.5納米的橫向和軸向分辨率,其圖像拍攝速率為200幀/秒,X、Y、Z互相成像速度為400赫茲,可對生物大分子進(jìn)行0.1PN作用力分辨率的實(shí)時(shí)分析。
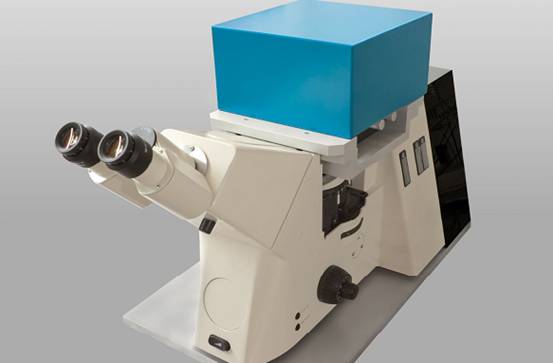
圖1 PicoTweezers裝配示意圖
系統(tǒng)工作原理:
分子之間的作用力是在三維方向上分布的,所以為了計(jì)算大分子之間產(chǎn)生的各種作用力,需要在X、Y、Z三個(gè)維度上進(jìn)行作用力的**檢測,并且要求在這三個(gè)維度的測量空間比較大,以實(shí)現(xiàn)實(shí)驗(yàn)的自由度和多目的性。
PicoTweezers系統(tǒng)使用視頻分析系統(tǒng)(右下圖)作為粒子追蹤、檢測和力測量的系統(tǒng),該系統(tǒng)雖然相對復(fù)雜,但模塊化的配置和穩(wěn)定的組裝賦予了該系統(tǒng)易于使用的特征。
在測量的過程中,被光阱捕獲的顆粒會(huì)在三個(gè)方向上對光進(jìn)行阻擋,導(dǎo)致光線偏轉(zhuǎn),PicoTweezers的視頻分析系統(tǒng)通過獲取偏轉(zhuǎn)的圖像來分析作用力的變化,進(jìn)而可以分析分子之間作用力的變化。由于該系統(tǒng)非常穩(wěn)定,且不需要校準(zhǔn)、沒有試驗(yàn)和空間的限制,所以該系統(tǒng)應(yīng)用領(lǐng)域非常廣泛,是生物學(xué)、分子生物學(xué)、材料學(xué)研究者在大分子生物力學(xué)研究中的***實(shí)驗(yàn)儀器之一。
系統(tǒng)擁有自動(dòng)、簡單和可靠的力校準(zhǔn)系統(tǒng)
該系統(tǒng)在三維空間中的力校準(zhǔn)是通過斯托克斯阻力法移動(dòng)3D-壓電平臺周邊的介質(zhì)來進(jìn)行的。在具體的應(yīng)用中,作用力的檢測是通過基于視頻的Allan方差分析和校準(zhǔn)途徑來實(shí)現(xiàn)的,其過程主要是通過對微小粒子的波動(dòng)進(jìn)行圖像記錄和分析,在此過程中,不需要施加任何摩擦力。在實(shí)驗(yàn)過程中,斯托克斯方法和Allan方差都不需要對光阱剛度k進(jìn)行判定,即使需要,視頻分析軟件可以自動(dòng)進(jìn)行計(jì)算。
系統(tǒng)的計(jì)算原理
系統(tǒng)擁有兩臺高速數(shù)碼照相機(jī)。**臺照相機(jī)對光學(xué)阱捕獲的粒子的周圍區(qū)域進(jìn)行成像,**臺高速CMOS照相機(jī)對被放大的粒子大圖像進(jìn)行測量。相關(guān)的關(guān)鍵會(huì)實(shí)時(shí)分辨并鎖定每個(gè)幀圖像的邊緣部分,確定出圖像的灰階和合適的范圍,以此來確定或獲取粒子的直徑。
當(dāng)外在的作用力施加在粒子上面時(shí)導(dǎo)致粒子在Z-軸上發(fā)生位移時(shí),粒子的直徑會(huì)發(fā)生變化,軟件會(huì)將這種變化通過Z-軸的作用力表現(xiàn)出來。而粒子受到水平方向上的作用力時(shí),僅僅改變圖像的圓心的位置,但是其直徑并不會(huì)改變。這樣通過獲取圖像的位置的變化就可以分析粒子納米級別的水平方向受到的作用力,進(jìn)而分析出粒子在X、Y軸上所受到的作用力。
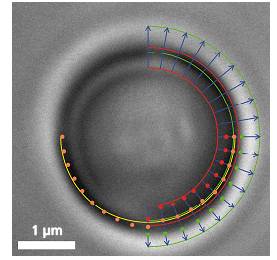
粒子在Z-軸上作用力會(huì)改變圖像的直徑,在X、Y軸上所受到的作用力會(huì)改變圖像的圓心位置
力測量的三種方式對比:
左邊:是傳統(tǒng)光鑷的監(jiān)測方法。激光通過捕獲粒子,然后收集前向散射光并投射到探測器感應(yīng)粒子的偏轉(zhuǎn)。由于獲取圖像之前需要設(shè)置冷卻器以進(jìn)行**地調(diào)節(jié),這種設(shè)置獲取的圖像容易漂移和錯(cuò)位,導(dǎo)致誤差;
中間:從顆粒被捕獲的目標(biāo)收集背散射光,入射激光設(shè)置分光器并投射到檢測器。這種方式測量的量程大,同樣可以建立基于視頻的檢測方法,但**度不夠。
右邊:這是PicoTweezer的檢測方法,同樣也是通過設(shè)置并收集背散射光,然后通過分光器并投射到檢測器。但是PicoTweezer系統(tǒng)采用了高靈敏度的CMOS圖像傳感器進(jìn)行處理,并添加一組聚焦透鏡進(jìn)行圖像聚焦,從而可以實(shí)現(xiàn)對納米粒子圖像的更**測定。其建立的基于圖像的檢測方法允許粒子更高的多樣性,數(shù)據(jù)吞吐速度更快,分析效果更**。采用這種方法的優(yōu)勢之一是激光器和光阱之間的光學(xué)路徑無需對檢測器進(jìn)行校準(zhǔn)或調(diào)整,對于被監(jiān)測的對象粒子的直徑和移動(dòng)可以被長期地監(jiān)測而不會(huì)發(fā)生漂移。
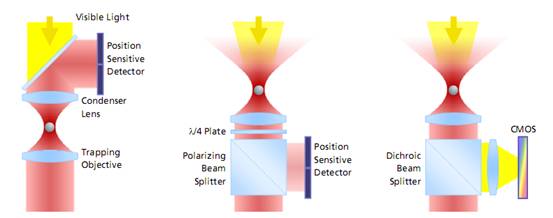
圖 光鑷子測量分子力的三種方式對比
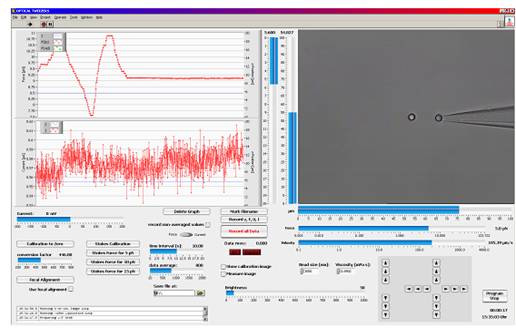
圖PicoTweezers軟件工作界面
儀器亮點(diǎn)
1)定量在三維方向?qū)崿F(xiàn)0.1 PN分辨率下的3D測量
2)*大光阱捕獲力可在1 W光纖激光器下達(dá)到400 PN
3)通過光鑷實(shí)現(xiàn)對捕獲對象精度為納米級別的操控
4)擁有緊湊、超穩(wěn)定模塊化系統(tǒng)
5)可編程的LabVIEW?軟件界面
6)不需要檢測校準(zhǔn),軟件計(jì)算非常容易
儀器應(yīng)用范圍
1.)單分子與活細(xì)胞的操控和分析
2)高分子彈性分析、微流控分析
3)分子相互作用、納米孔分析
應(yīng)用案例
1)單分子的捕獲及分析
單個(gè)DNA鏈通過兩端的官能基團(tuán)固定在兩個(gè)微珠之間。其中的一個(gè)微珠被玻璃微吸管固定,另外一個(gè)微珠被光鑷俘獲,通過移動(dòng)壓電平臺增加珠之間的距離引起的受控DNA的機(jī)械張力。作為響應(yīng)的分子的力 - 延伸曲線表現(xiàn)出DNA特定的機(jī)械性能,結(jié)果可以計(jì)算DNA的熵彈性,DNA的一個(gè)過渡延伸平臺區(qū)和熔融過渡區(qū)域。
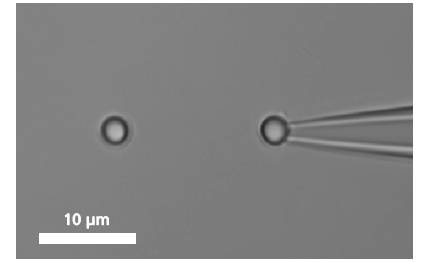
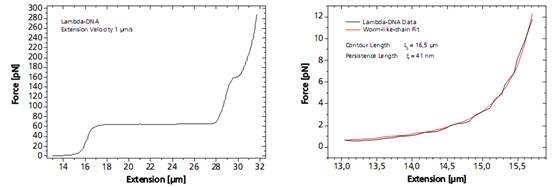
圖DNA分子的捕獲及分析圖譜
2)單個(gè)DNA鏈的易位過程分析
PicoTweezers可用于納米孔分子生物學(xué)的研究,他們迅速演變成單分子檢測領(lǐng)域一個(gè)非常新穎的技術(shù)。當(dāng)單個(gè)DNA分子或DNA-蛋白復(fù)合物通過納米孔時(shí),這個(gè)過程可以被PicoTweezers監(jiān)測并分析,相關(guān)數(shù)據(jù)可以確定DNA的易位動(dòng)力學(xué)和DNA上結(jié)合的蛋白質(zhì)的位置。
納米孔測量的優(yōu)勢主要表現(xiàn)在:(1)可在非常低的濃度和很小的樣品體積下測定目標(biāo)分子;(2)可同時(shí)進(jìn)行基因與生物標(biāo)志物的篩選;(3)由于不需要進(jìn)行放大及轉(zhuǎn)化,分析測量的速度將很快且費(fèi)用低廉。
當(dāng)捕獲的的粒子上的DNA接近納米孔(至5微米的距離)時(shí),帶負(fù)電荷的DNA通過DNA骨架的靜電力立即進(jìn)入納米孔中,這種效果可以通過監(jiān)測的力信號得到??梢钥吹竭@種作用力在到達(dá)一定穩(wěn)定值后突然變化,顯示出DNA的易位過程。系統(tǒng)所測得的力取決于施加的電壓以及納米孔的直徑。當(dāng)整個(gè)DNA鏈與納米孔另外一端的距離為10.5微米時(shí),表明DNA鏈被拉出孔,所述力降回到零。
目前PicoTweezers可結(jié)合各個(gè)實(shí)驗(yàn)室已構(gòu)建的納米尺度裝置對單分子進(jìn)行分析。包括生物納米孔(通道) (由各類蛋白質(zhì)分子鑲崁在磷脂膜上組成)、固態(tài)納米孔(通道)(包括各種硅基材料、SiNx、碳納米管、石墨烯、玻璃納米管等)及兩類相結(jié)合的雜化納米孔(通道)。
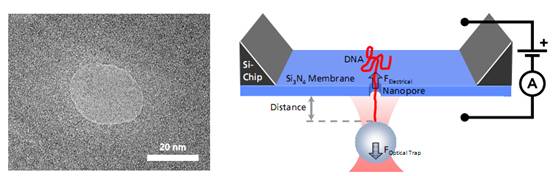
圖 DNA的易位過程分析圖譜
3)單個(gè)DNA結(jié)合蛋白分析
當(dāng)結(jié)合到DNA鏈上的單個(gè)過氧氧化還原酶通過納米孔過程中,會(huì)發(fā)生非對稱的力的信號。這種信號可以作為蛋白質(zhì)在DNA上的結(jié)合位點(diǎn)的無標(biāo)記的定位信息。相關(guān)的力學(xué)信息結(jié)合DNA和蛋白質(zhì)的特征,可以進(jìn)一步得出其蛋白質(zhì)與DNA的結(jié)合作用力來源于蛋白質(zhì)與DNA骨架電荷的作用力。當(dāng)單個(gè)DNA結(jié)合蛋白通過納米孔時(shí),DNA與結(jié)合蛋白之間的靜電引力減少,有助于分子通過微孔。
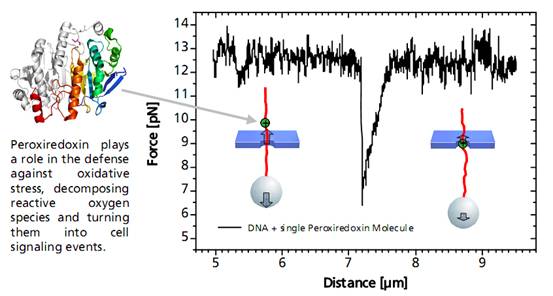
圖單個(gè)DNA結(jié)合蛋白分析圖譜
參考文獻(xiàn)
-
S. Knust, A. Spiering, H. Vieker, A. Beyer, A. G?lzh?user, K. T?nsing, A. Sischka and D. Anselmetti Video-Based and Interference-Free Axial Force Detection and Analysis for Optical Tweezers Review of Scientific Instruments, 83, 103704 (2012)
-
Spiering, S. Getfert, A. Sischka, P. Reimann and D. Anselmetti Nanopore Translocation Dynamics of a Single DNA-Bound Protein Nano Letters, 11, 2978 (2011)
-
Sischka, A. Spiering, M. Khaksar, M. Laxa, J. K?nig, K.J. Dietz and D. Anselmetti Dynamic Translocation of Ligand-Complexed DNA Through Solid-State Nanopores with Optical Tweezers Journal of Physics - Condensed Matter, 22, 454121 (2010)
-
Kleimann, A. Sischka, A. Spiering, K. T?nsing, N. Sewald, U. Diedrichsen and D. Anselmetti Binding Kinetics of Bisintercalator Triostin A with Optical Tweezers Force Mechanics Biophysical Journal, 97, 2780 (2009)
-
Pla, A. Sischka, F. Albericio, M. Alvarez, X. Fernandez-Busquets and D. Anselmetti Optical-Tweezers Study of Topoisomerase Inhibition Small, 5, 1269 (2009)
-
Sischka, C. Kleimann, W. Hachmann, M.M. Sch?fer, I. Seuffert, K. T?nsing and D. Anselmetti Single Beam Optical Tweezers Setup with Backscattered Light Detection for Three-Dimensional Measurements on DNA and Nanopores Review of Scientific Instruments, 79, 063702 (2008)
-
Anselmetti, N. Hansmeier, J. Kalinowski, J. Martini, T. Merkle, R. Palmisano, R. Ros, K. Schmied, A. Sischka and K. T?nsing Analysis of Subcellular Surface Structure, Function and Dynamics Analytical and Bioanalytical Chemistry, 387, 83 (2007)
-
Sischka, K. T?nsing, R. Eckel, S.D. Wilking, N. Sewald, R. Ros and D. Anselmetti Molecular Mechanisms and Kinetics between DNA and DNA Binding Ligands Biophysical Journal, 88, 404 (2005)
|
英文介紹Introduction
Our Optical Tweezers System Provides:
-
Quantitative 3D Force Measurements with 0.1 pN Resolution
-
Achievable Trapping Force of 400 pN with 1 W Fiber Laser
-
Manipulation of Trapped Objects with Nanometer Precision
-
Compact and Ultrastable Modular System
-
Programmable LabView? Software Interface
-
Easy-to-use Force Calibration without Detector Alignment
-
Scope of Applications:
-
from Single Molecules to Living Cells
-
from Polymer Elasticity to Microfluidics
-
from Molecular Interactions to Nanopores
Optical Trapping and Force Measurement
Optical tweezers are used to trap and actively manipulate microscopic objects. They also offer a vast area of applications by measuring forces applied to trapped objects.
The Optical Trap
Microscopic objects - like individual nano- or microparticles, cells, cell compartments, single or clustered molecules - can be trapped securely inside the center of a strongly focused laser beam.
When an external forces is acting on the trapped object, it deflects from the center of the trap as the deflection x depends linearly on trap stiffness k and force F.
Lateral and axial forces acting on a trapped particle
Forces
A trapped particle experiences various external forces. Atoms or molecules of the surrounding medium induce Brownian motion in all three dimensions, depending on temperature, viscosity and the presence of obstacles in the vicinity. Macroscopic fluid movements cause a drag force. Electric fields and bulk or surface charges may generate electrophoretic or electroosmotic forces.
Particularly, single molecules can induce forces of broad variety and magnitude while bound to the trapped object. On the other hand, the application of a force generated by an optical trap to a single molecule will gain vast insight into the molecular structure and elasticity, binding properties and kinetics.
Deflection is the Essence
Generating and metering various forces requires a reliable force measurement capability in all three dimensions to allow for a maximum degree of experimental freedom and versatility. Therefore, force detection is accomplished by precisely measuring the deflection of the trapped particle in each direction.
The PicoTweezers system utilizes a sophisticated and easy-to-use video analysis (right image below) for particle tracking, detection and force measurements. It offers the largest field of application since it clears common calibration difficulties, system instabilities, as well as experimental and spatial restrictions.
The evolution of force measurement. Left: Laser light passing trough the trapped particle (forward scattered light) is collected and projected onto a detector sensing the particle’s deflection. The condenser in close proximity to the trapping objective needs to be precisely adjusted. It is susceptible against drift and misalignment and limits the experimental space. Center: Backscattered light from the particle is collected by the trapping objective, separated from the incident laser light and projected onto the detector. This extremely robust setup allows high experimental freedom – the same applies for video-based detection method in the right image, where no detector alignment is required, too. In addition, a high diversity of trapped particles can be video-analyzed and measured.
Video Detection and Analysis
Video-based force detection is easy to calibrate and provides an alignment-free and unsusceptible method for all force measurements in three dimensions. It is embedded into the LabView? platform.
The Principle
Video frame of a trapped microbead with various overlaid detection lines
In addition to a video camera imaging the surrounding area of the optical trap, a second high-speed CMOS camera simultaneously surveys the magnified image of the trapped particle. The software searches for specific edges (as shown in the upper right quadrant of the image) in each frame and in real-time, determines gradients (lower right quadrant) and fits a circle (lower left quadrant) which correlates to the apparent particle diameter.
If an external axial force is acting on the particle, its apparent diameter changes, which the software translates into a z-force. Lateral forces only shift the center of the particle. These lateral deflections in the order of nanometers are then translated into x- and y-forces.
Easy and Reliable Force Calibration
LabView? based trapping, calibration and measurement software
Force calibration in three dimensions is conducted by moving the surrounding medium via the piezo stage using Stokes’ drag force law.
For specific applications, video-based force detection utilizes Allan Variance analysis and calibration. Here, smallest particle fluctuations are recorded and analyzed without the need of applying any frictional force. Both Stokes’ method and Allan Variance do not require the determination of the trap stiffness k, though the video-analysis software can calculate it if desired.
Benefits of Video-Based Force Detection
There is no need of detector alignment or adjustment in the beginning or during experimentation because the CMOS camera providing data for video detection and analysis is integrated into the optical pathway between laser and optical trap. Video detection is unsusceptible to disturbing particles that occasionally may be trapped together with the measured object. Since the diameter of the trapped object is permanently monitored, further particles of interest can be trapped and compared with previous ones. Specifically tailored Allan Variance for video analysis is a powerful calibration tool for experiments that take place in an environment that prevents other calibration or analysis methods. When trapping particles close to interfaces (bottom or ceiling of sample chamber, artificial or biological membranes, etc.), video analysis delivers an interference-free force signal.
Detection Tandem
Optionally, PicoTweezers can be equipped with additional backscattered light detection capability for simultaneous measurements or as stand-alone method, if experiments need to be conducted in absence of light or if particle fluctuations must be analyzed with highest sample rate in the kHz range.
Applications — Single Molecules and Polymer Elasticity
The elastic behavior of a single DNA-strand in absence or in presence of binding ligands can be reliably measured. Theoretical polymer models that are fitted to the results will deliver parameters, which characterize the polymer elasticity.
Grabbing a Single Molecule
A single DNA is immobilized between two microbeads.
To bind a single DNA-strand between two coated microbeads, it has to be properly functionalized on both ends. Thus, it can be immobilized between two beads, of which one is optically trapped and the other is held on the tip of a micropipette. Increasing the distance between the beads by moving the piezo stage induces a controlled mechanical tension to the DNA.
As a response, the force-extension curve of the molecule exhibit characteristic mechanical properties, such as an entropic elasticity, an overstretching plateau and a melting transition region.
Left: Force response of a single 48502 base pair long DNA molecule of bacteriophage lambda. In the force range up to 10 pN the entropic regime determines the elastic behavior of the molecule, whereas around 65 pN the characteristic overstretching transition occurs. The nature of this phenomena remains controversial, as well as for a less pronounced transition at 160 pN. Right: Fitting the Worm-like-chain model to the entropic regime yields two intrinsic elasticity parameters. For example, the persistence length strongly depends on salt concentration and on the presence of DNA-binding ligands.
DNA as Sensor for Foreign Molecules
The DNA strand can serve as a host for a variety of different molecules, such as small intercalators, groove-binders, proteins, enzymes or molecular motors.
The binding event of a single or a multitude of ligands can change the elastic response more or less significantly. As an example, the force curve of a DNA is shown in presence of the antibiotic distamycin-A that attaches to the minor groove of the DNA strand while stabilizing it and helping to resist the overstretching. On the other hand, diazoniapentaphene as an intercalator increases both contour and persistence length and renders the overstretching plateau to disappear.
Small or large variations in the elastic response of a DNA-molecule in the presence of binding ligands can be measured.
Applications — Translocation through Nanopores
Nanopores play a major role in biology and they rapidly evolved into a new and promising technique in single-molecule detection. The controlled threading of a single DNA molecule or a DNA-protein complex into a nanopore allows investigation of the translocation dynamics and a localization of the bound protein.
Left: TEM image of a solid-state nanopore drilled with a focused ion beam machine into a Si3N4membrane that serves as model system to study single molecule translocations. Right: Experimental setup of a DNA translocation measured with optical tweezers. When applying a voltage across the membrane, a single DNA molecule immobilized on a trapped microbead translocates through the pore. The electrostatic force acting on the molecule and the distance between bead and nanopore can be precisely measured.
Translocating a Single DNA Strand
When the DNA on the trapped bead approaches the nanopore (to a distance of 5 μm) it is immediately threaded into the pore by electrostatic forces acting on the negatively charged DNA backbone. This effect can be monitored as an abrupt step of the force signal to a certain value, which remains constant even when retracting the bead. The measured force depends on the applied voltage, as well as on the diameter of the nanopore.
When the entire DNA strand with an end-end-distance of 10.5 μm is pulled out of the pore, the force drops back to zero.
Controlled DNA threading into a 55 nm solid-state nanopore with an applied voltage of 50 mV.
Single DNA-Bound Protein
A distinct asymmetric force signal occurs when a single peroxiredoxin molecule bound to the DNA stand is actively pulled through the pore. This effect serves as a label-free localization of the protein binding site.
It can be understand as the result of an effective positive charge of the protein counteracting the negative DNA backbone charge and reducing the electrostatic force.
Characteristic force signal of a single peroxiredoxin molecule bound to a DNA strand when both are translocated through a 35 nm nanopore.
We Design and Build Your Optical Tweezers.
PicoTweezers is a stand-alone system, that can also be customized to your Zeiss Axiovert, Axio Observer A1 or D1. It will be equipped with a 1 W or 5 W IR fiber laser for highest spatial trap stability yielding a trapping force of at least 400 pN or 2 nN, respectively.
The 3D-piezo stage enables nanometer resolution in a range of 200 μm in x and y, as well as 20 μm in z-direction. Video-analysis can achieve a lateral and axial resolution of at least 2.5 nm, which results in a force resolution of 0.1 pN with a frame rate of 200 and 400 Hz in z- and x,y-direction, respectively
References
-
S. Knust, A. Spiering, H. Vieker, A. Beyer, A. G?lzh?user, K. T?nsing, A. Sischka and D. Anselmetti
Video-Based and Interference-Free Axial Force Detection and Analysis for Optical Tweezers
Review of Scientific Instruments, 83, 103704 (2012)
-
A. Spiering, S. Getfert, A. Sischka, P. Reimann and D. Anselmetti
Nanopore Translocation Dynamics of a Single DNA-Bound Protein
Nano Letters, 11, 2978 (2011)
-
A. Sischka, A. Spiering, M. Khaksar, M. Laxa, J. K?nig, K.J. Dietz and D. Anselmetti
Dynamic Translocation of Ligand-Complexed DNA Through Solid-State Nanopores with Optical Tweezers
Journal of Physics - Condensed Matter, 22, 454121 (2010)
-
C. Kleimann, A. Sischka, A. Spiering, K. T?nsing, N. Sewald, U. Diedrichsen and D. Anselmetti
Binding Kinetics of Bisintercalator Triostin A with Optical Tweezers Force Mechanics
Biophysical Journal, 97, 2780 (2009)
-
D. Pla, A. Sischka, F. Albericio, M. Alvarez, X. Fernandez-Busquets and D. Anselmetti
Optical-Tweezers Study of Topoisomerase Inhibition
Small, 5, 1269 (2009)
-
A. Sischka, C. Kleimann, W. Hachmann, M.M. Sch?fer, I. Seuffert, K. T?nsing and D. Anselmetti
Single Beam Optical Tweezers Setup with Backscattered Light Detection for Three-Dimensional Measurements on DNA and Nanopores
Review of Scientific Instruments, 79, 063702 (2008)
-
D. Anselmetti, N. Hansmeier, J. Kalinowski, J. Martini, T. Merkle, R. Palmisano, R. Ros, K. Schmied, A. Sischka and K. T?nsing
Analysis of Subcellular Surface Structure, Function and Dynamics
Analytical and Bioanalytical Chemistry, 387, 83 (2007)
-
A. Sischka, K. T?nsing, R. Eckel, S.D. Wilking, N. Sewald, R. Ros and D. Anselmetti
Molecular Mechanisms and Kinetics between DNA and DNA Binding Ligands
Biophysical Journal, 88, 404 (2005)